- Featured Articles
- Long-Term Results: Chlorinated Solvent Treatment Using EZVI Injection with Supplemental Bioremediation and Bioaugmentation
- Bench-Scale Study: Nanoscale ZVI/Pd and GAC Composite in Sediment Cap Used to Dechlorinate PCBs
- Resources
Download This Issue in Adobe PDF® Format
(1.04MB/7pp/PDF)
This issue of Technology News & Trends highlights the use of nanotechnologies to chemically reduce chlorinated volatile organic compounds (CVOCs), metals and metalloids such as chromium and arsenic, and persistent organic compounds contaminating groundwater, soil, or sediment. Nanoscale materials typically used for remediation involve nanoscale zero-valent iron (ZVI), bimetallic nanoscale particles, or emulsified zero-valent iron (EZVI). The U.S. Environmental Protection Agency's National Risk Management Research Laboratory and other agencies or organizations are researching remediation efficacy as well as fate, transport, and toxicity of these and other nanoscale materials with potential to adsorb or destroy contaminants as part of in situ or ex situ processes.
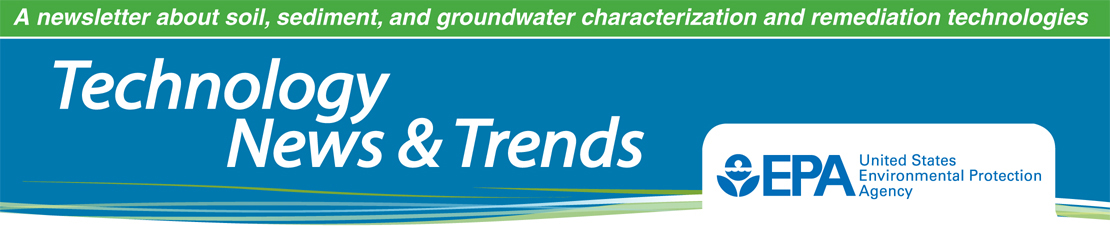
Long-Term Results: Chlorinated Solvent Treatment Using EZVI Injection with Supplemental Bioremediation and Bioaugmentation
Contributed by Regina Dixon Butler, Patrick Air Force Base; John Armstrong, Florida Department of Environmental Protection; Deda Johansen, Jacobs Engineering Group
Remediation of contaminated groundwater at Patrick Air Force Base's OT030 site combined the application of emulsified zero-valent iron (EZVI) with enhanced biodegradation. The impacted area encompasses several facilities where past cleaning and maintenance operations released chlorinated solvents to groundwater, creating an approximate 36-acre contaminant plume. Investigations indicated a primary source area covering 0.12 acres where trichloroethene (TCE) exceeded 100 milligrams per liter (mg/L). Fate and transport modeling predicted that natural degradation would take more than 240 years. EZVI injection combined with emulsified vegetable oil (EVO) application and bioaugmentation were selected to accelerate this process. Cleanup goals for the corrective measures included reducing total plume mass 50% within 25 years, 75% within 40 years, and 100% (achieving state groundwater cleanup target levels [GCTLs]) within 80 years.
Site soil consists of fine- to medium-grained sand with silt and shell fragments to a depth of approximately 48 feet below ground surface (bgs). Chlorinated volatile organic compound (CVOC) contamination is limited to this upper layer of the surficial aquifer due to an underlying clay layer approximately 20 feet thick. The CVOC plume extends downgradient and nearly reaches the Banana River Lagoon (BRL), an Outstanding Florida Water. Factors influencing selection of a suitable remediation technology included proximity to the BRL; the presence of dormitories, offices, and mission-critical operations; and numerous underground utilities (Figure 1).
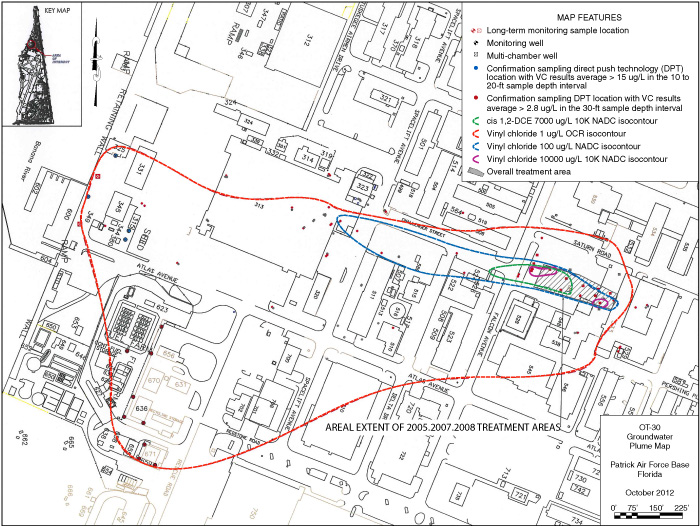
Figure 1. CVOC source area and plume at OT030 as of 2012.
Design for full-scale EZVI application at OT030 was based on results of a National Aeronautics and Space Administration field demonstration at Cape Canaveral Air Force Station, Florida. [For more information about the demonstration, see the September 2005 issue of Technology News and Trends]. EZVI relies on the use of nanoscale or microscale grains of iron mixed in vegetable oil to create an emulsion that promotes contact between the iron and the chlorinated solvent and brings about direct dechlorination. The vegetable oil component also acts as a substrate for microbial growth, providing additional long-term treatment by enhancing reductive dechlorination via native or augmented communities of bacteria.
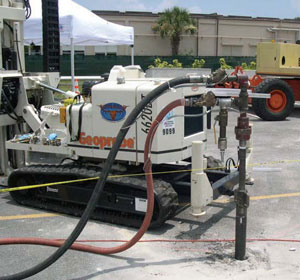
Figure 2. Pneumatic and product hoses attached to a 360°-rotatable injection nozzle on a direct push rig.
Groundwater treatment involved multiple injections between 2005 and 2008. Aggressive source reduction was the initial objective, with EZVI as the primary treatment agent. The emulsion was injected pneumatically to create a fluidized subsurface lens and 6-foot radius of influence (ROI) (Figure 2). A proprietary mixture of nanoscale and microscale ZVI, including ZVI measuring 0.2 µm, 1.5 µm, and 3.0 µm, was used to benefit from the greater surface area provided by nanoscale ZVI and the lower cost and easier availability of microscale ZVI.
Over five months in 2005, a total of 60,100 gallons of EZVI was injected sequentially at 36 points with a target depth interval of 26-42 feet bgs. To create a zone of enhanced biological reduction, 9,350 gallons of EVO (as a substrate for microbial growth) were injected in six points on the downgradient side. A microbial consortium (KB-1™) also was added.
The highest TCE concentration in groundwater decreased 98% (from 350 mg/L to 4.5 mg/L) within one year after EZVI injection completion. As anticipated, TCE breakdown products cis-1,2-dichloroethene (DCE) and vinyl chloride (VC) increased. Concentrations of the three contaminants continued to exceed the Florida GCTLs of 3, 70, and 1 µg/L, respectively.
In light of the substantial degradation of TCE by EZVI, further use of EVO and bioaugmentation offered cost-effective treatment for a plume now consisting mainly of breakdown products. A follow-up injection of 82,876 gallons of EVO was conducted in July 2007 to replenish substrate in the previously treated area and expand the area of enhanced reductive dechlorination. The 53 injection points also received 402 liters of the microbial consortium. EVO quantities were adjusted based on contaminant concentrations, varying from 4 to 8% pore space saturation. Conventional drilling equipment was employed during this injection since recent lessons learned at other local sites demonstrated a sufficient ROI without more costly pneumatic fracturing. Associated savings allowed a third treatment event. In 2008, semi-permanent injection ports were installed along the downgradient axis of the source area and 12,000 gallons of EVO mixture were introduced to maintain bioremediation activity.
Sixteen post-injection sampling events occurred between February 2006 and May 2012 at selected wells throughout the plume. Initial monitoring was robust and focused on the treated areas. More detailed five-year monitoring data were collected at 39 monitoring wells and 35 ports from 13 multi-chamber wells to ensure the conceptual site model remains accurate.
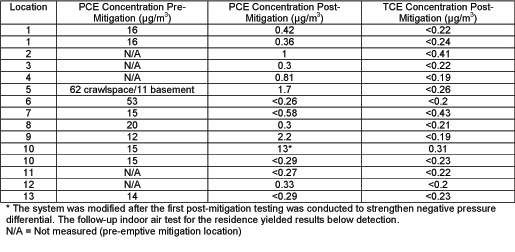
Figure 3. CVOC trends in OT030 well with highest pre-treatment TCE concentration, at 35 feet bgs.
With one exception possibly caused by matrix interference, no TCE concentrations above the GCTL were detected in the two most recent sampling events, and daughter product concentrations were also reduced to a lesser extent.. Maximum DCE and VC concentrations were 31,000 µg/L and 12,000 µg/L, respectively, as compared to baseline (pre-treatment) source area samples containing up to 90,000 µg/L DCE and 24,500 µg/L VC. Total CVOC mass in the source area declined nearly 89% as of October 2011, to about 886 µmol/L. In the well with the site�s highest TCE concentrations prior to treatment, an estimated 99% reduction in TCE concentrations was achieved (Figure 3).
Data collected from 64 monitoring wells demonstrate reductive geochemical conditions in the subsurface. Several geochemical indicators demonstrate favorable conditions for anaerobic degradation, including a mean ORP of -226 mV, mean pH of 7.05, and dissolved oxygen concentration of 0.44 mg/L.
Evaluation of other geochemical indicators also suggests that biodegradation is occurring. Favorable trends include increasing dissolved methane (from an average of 113.4 µg/L prior to injections to 2,783 µg/L in October 2011), decreasing sulfate (from 985 mg/L to 551 mg/L), increasing sulfide (from 0.685 mg/L to 31 mg/L), decreasing nitrate (from 0.424 µg/L to 0.07 µg/L), increasing nitrite (from 0.15 µg/L to 1.03 µg/L), increasing carbon dioxide (84.2 µg/L to 800 µg/L), and increasing alkalinity (from 470 µg/L to 792 µg/L). In addition, Dehalococcoides screening indicates increasing counts of the gene capable of dechlorinating VC (VC reductase). October 2011 data across 12 wells yielded an average gene count of 34,222,182 cells/L, consistent with the 10' value typically considered effective.
MODFLOW-2000 and RT3D software were used to update the fate and transport model, which now predicts that GCTLs will be attained in 2080. Continued semi-annual sampling is planned at selected monitoring wells indefinitely to monitor natural attenuation and evaluate the need for additional groundwater treatment due to potential cis-DCE degradation "stall." In November 2012, an additional EVO injection was completed to provide continued substrate for biodegradation in and immediately downgradient from the previous treatment area, where cis-DCE concentrations continue to exceed 7,000 µg/L.
Costs for treatment and annual monitoring from 2004 (baseline sampling) to the October 2012 monitoring event total approximately $6.5 million. Lessons at OT030 paved the way for treatment at several other sites. Most recently, an EZVI application at Cape Canaveral Air Force Station�s Hangar K site used an adjustable pore space dilation technique developed during EVO injections at OT030 to treat two TCE source areas with 61,645 gallons of EZVI.
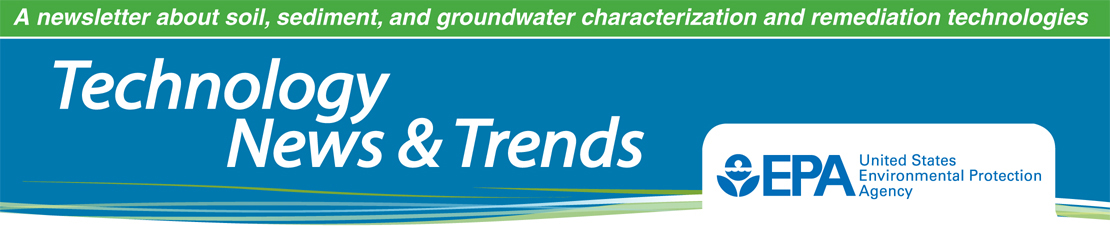
Bench-Scale Study: Nanoscale ZVI/Pd and GAC Composite in Sediment Cap Used to Dechlorinate PCBs
Contributed by Dr. Souhail Al-Abed (U.S. EPA National Risk Management Research Laboratory)
The U.S. Environmental Protection Agency (EPA) National Risk Management Research Laboratory (NRMRL) developed a laboratory-scale nanoscale zero-valent iron (ZVI) and palladium application in 2008-2009 to facilitate cleanup of sediments contaminated with polychlorinated biphenyls (PCBs). The technology employs granular activated carbon (GAC) composite impregnated with 6- to 12-nanometer (nm) reactive iron (Fe) particles and small amounts of 2- to 3-nm palladium (Pd) to form a reactive activated carbon (RAC) that actively adsorbs and reductively dechlorinates PCBs and other chlorinated compounds. NRMRL is collaborating with the University of Texas at Arlington, Stanford University, Australia�s Commonwealth Scientific and Industrial Research Organisation, the University of South Wales, and the and N. D. Zelinsky Institute of Organic Chemistry, Russian Federation, to perform additional bench-scale comparative reductive dechlorination studies and field tests examining the effectiveness of RAC in natural systems.
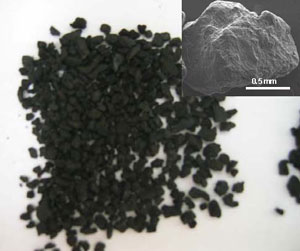
Figure 1. RAC consisting of Fe/Pd on GAC.
The first step of PCB treatment is its desorption from the sediment matrix to aqueous-phase. GAC can facilitate desorption of PCBs in a stable sediment matrix but will not degrade contaminants. Nanoscale bimetallic systems can be effective in dechlorinating contaminants but depend on the passive contact of the materials with PCBs. By synergistically combining the two remediation technologies, RAC (Figure 1) takes advantage of the high adsorptive capacity of GAC and actively attracts hydrophobic PCBs present in the sediment matrix, while the Fe/Pd facilitates the physical reductive dechlorination reaction.
To synthesize RAC (Figure 2), mesoporous GAC is introduced as support material for Fe particles to disperse and as an adsorptive material for PCBs to contact with Fe particles. The Fe surface is then modified with a discontinuous layer of noble catalytic metal Pd to facilitate electron generation for PCB dechlorination. Specifically, the process involves melting relevant iron salt at 55-60°C, mixing with GAC, drying at 60-70°C and calcining at 300�C in furnace to obtained ferric oxide (Fe2O3)/GAC composite. To reduce Fe2O3 to ZVI, sodium borhydride (NaBH4) is added to GAC/Fe2O3 suspended in methanol/water (30:70 by volume) at a pH of 6.5. GAC/ZVI is then recovered and washed with methanol to remove free ZVI and other impurities and dried at room temperature. Pd is added by mixing the as prepared composite with relevant palladium salt. Finally, the GAC/ZVI/Pd (RAC) composite is recovered, washed, dried, and stored prior the use. Samples are sieved at the end of each procedure to remove grains smaller than 0.85 mm.
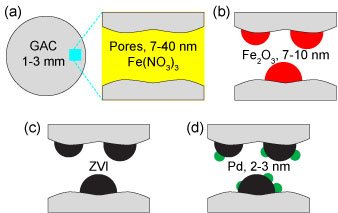
Figure 2. RAC synthesis process: (a) mesoporous GAC is embedded with ferric nitrate nonahydrate (Fe(NO3)3·9H2O); (b) GAC is calcined to transform Fe(NO3)3 to ferric oxide (Fe2O3); (c) Fe2O3 is reduced to ZVI; (d) Pd is added and Fe and Pd particles are nanoscaled.
A 2009 NRMRL study of RAC involved batch reactions testing the adsorption and dechlorination reaction kinetics of 2-chlorobiphenyl (2-ClBP). The mono-PCB congener was chosen for the study due to its high water solubility, its persistence for hydrodechlorination, and its transformation to a single dechlorination product, biphenyl (BP), which facilitates a more straightforward evaluation of process kinetics. During the study, RAC and GAC (control) were each added at concentrations of 5, 20, and 100 grams per liter (g/L) to 10 mL of 4 milligrams per liter (mg/L) 2-ClBP. The solutions were constantly agitated until removed for examination at 0, 4, 8, 24, 48, 120, and 240 hours. After 48 hours of reaction, most of the 2-ClBP was gone from the liquid phase while an insignificant amount of BP remained in both the RAC and GAC solutions. For the GAC solutions, loss of 2-ClBP from the liquid phase was solely attributed to its adsorption to the activated carbon. In the RAC solutions, the 2-ClBP was found to first adsorb to the activated carbon and then immediately undergo reductive dechlorination. Reaction kinetics indicated that adsorption of 2-ClBP to the RAC is slower than to GAC because RAC has fewer adsorptive sites.
High recovery of 2-ClBP was achieved with both the GAC and RAC. In the GAC (no dechlorination), 93-100% of 2-ClBP was removed from solution at a stable rate irrespective of the contaminant load levels. Removal of 2-ClBP from solution and subsequent dechlorination by RAC varied with contaminant load. At a loading concentration of 5 g/L, 86% of 2-ClBP was removed from solution and dechlorinated within two days. At concentrations of 20 and 100 g/L, nearly complete dechlorination of 2-ClBP was achieved within one day (Figure 3).
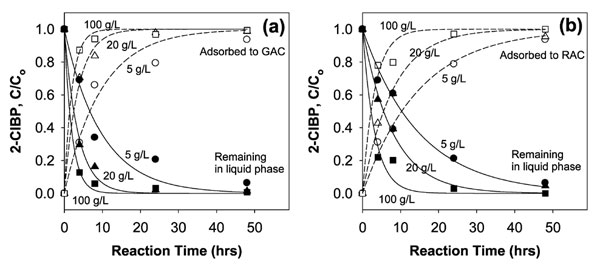
Figure 3. Adsorption of 2-ClBP with GAC (a) and RAC (b) at different loading rates.
Results from the laboratory study suggested that RAC pellets could potentially be used between thin geotextile membranes of sediment capping material, which would act as a barrier between contaminated sediment and water limiting contact between organic matter in the water column and PCBs, which have high affinity for organic substances. NRMRL is conducting additional bench-scale studies to examine the effects of reaction environments relevant in natural systems, such as initial concentration, pH, and co-existing natural organic matter and ionic species, on the implementation of RAC. The full-cycle transport, reaction, and fate of PCBs in sediment are also being examined to determine the role of organic carbon and aging.
RAC can be directly mixed with the contaminated sediment matrix for prompt sequestration of PCBs. A RAC cap can be installed horizontally to contain PCB-contaminated sites, while a RAC barrier can be installed vertically for flow-through treatment of contaminated groundwater. A field-scale application that uses a reactive cap and barrier composed of RAC is currently being tested at various locations in the Sydney Harbour to clean up PCB-contaminated sediment, and at Botany Bay in New South Wales to prevent migration of chlorinated hydrocarbons from a nearby industrial park.
In addition, joint work between University of Texas at Austin and the N.D. Zelinsky Institute of Organic Chemistry, Russian Federation, is being performed to examine reductive dechlorination of trichloroethene (TCE) and tetrachloroethene (PCE) and the stability of TCE and PCE adsorbed to the supported bimetallic nanoparticles as a function of particle type and reaction conditions. A major focus of the research is the longevity of the degradation process as a function of contaminant loading.
RAC studies are contributing to a new direction of research on the use of permeable reactive barriers in variety of hydrogeological settings. RAC also can treat coexisting contaminants, including halogenated compounds, and consequently provides effective tools to manage PCB-contaminated sites in a manner that gains regulatory acceptance. Further research can be initiated to better understand the transport, reaction, and fate of such contaminants on RAC when the technology is used as an environmental risk management option. To improve full-scale RAC application in the field, future NRMRL research is expected to focus on exploring the possibility of using more cost-efficient methods to reduce Fe to ZVI, more environmentally sustainable reducing reagents such as ascorbic acid, and less expensive metals such as copper and nickel.
RESOURCES
CLU-IN Website: Nanotechnology: Applications for Environmental Remediation
This remediation technology area of CLU-IN provides a compendium of guidance materials, application reports, and other information resources on naturally occurring and engineered materials containing an active component with submicron dimensions.
Upcoming Workshop: Applications of Nanotechnology for Safe and Sustainable Environmental Remediation
Government, industry, academic, and other partners have organized a national workshop to be held at Southeastern Louisiana University in Hammond, Louisiana, on June 5-7, 2013. The workshop offers a venue to develop ideas about designing guidelines, criteria, and work practices that support safe and sustainable nano-enabled environmental remediation, become acquainted with nanotechnology vendors and other stakeholders, and share case studies of nano-enhanced cleanup technologies. Results of the workshop are expected to help environmental cleanup practitioners anticipate, recognize, evaluate, control, and confirm the safe management of potential risks associated with occupational and environmental exposures to nanomaterials.
SERDP Demonstrations
- Application of Microarrays and qPCR to Identify Phylogenetic and Functional Biomarkers Diagnostic of Microbial Communities that Biodegrade Chlorinated Solvents to Ethene (ER-1587; January 2013 fact sheet and upcoming reports). This research established and applied a method of fluorescence-activated cell sorting combined with whole genome amplification and microarray techniques to examine dilute quantities of Dehalococcoides species without time-consuming culturing steps. The approach may be used to optimize in situ bioremediation technologies and provide biomarkers that could replace or supplement traditional diagnostics for biodegradation in anaerobic environments.
- A Portable Surface-Enhanced Raman Sensor for Real-Time Detection and Monitoring of Perchlorate and Energetics (ER-1602; January 2012 final report). Sensitive gold nanoparticle-based surface- enhanced Raman spectroscopy substrates were developed and integrated with a Raman analyzer (equipped with a near infrared laser and fiber-optic probe) to create a miniaturized field-deployable sensor that detects energetic and co-contaminants in groundwater and surface water. Oak Ridge National Laboratory and project partners demonstrated that the sensor could detect perchlorate, TNT, and RDX in the field at concentrations as low as 0.1 µg/L, 2.3 µg/L, and 0.12 mg/L, respectively.
Recent Publications
- Toxicity of Nano-Zero Valent Iron to Freshwater and Marine Organisms
University of California-Santa Barbara researchers recently tested whether three commercial forms of nanoscale ZVI (uncoated, organic coating, and iron oxide coating) applied for soil remediation are toxic to downstream freshwater and marine organisms. The research findings can be used to design a risk management strategy that arrests transport of injected nanoscale ZVI beyond the intended remediation area. - SETAC Technical Workshop Summary: Guidance on Passive Sampling Methods to Improve Management of Contaminated Sediments
In November 2012, the Society of Environmental Toxicology and Chemistry (SETAC) held a workshop to promote understanding of passive sampling methods (PSMs) and provide recommendations for their application in contaminated sediment assessment and management. The workshop focused on PSMs as a means to quantify the bioavailability of organics or metals, based on the diffusion and subsequent partitioning of contaminants from sediment to a reference sampling phase. Preliminary findings of the workshop and plans for developing related issue papers are now available in a SETAC technical workshop summary.
Contact Us:
Suggestions for articles in upcoming issues of Technology News and Trends may be submitted to
Linda Fiedler via email at fiedler.linda@epa.gov.
Past Issues:
Past issues of the newsletter are available at http://www.clu-in.org/products/newsltrs/tnandt/.
Archives | Subscribe | Change Your Address | Unsubscribe