Fractured Rock
Overview
Groundwater flow and the fate and transport of contaminants in fractured rock are influenced by the characteristics of the rock and thus differ from unconsolidated deposits (e.g., silt, sand, or gravel). Due to the differences, approaches to characterizing and remediating unconsolidated deposits may prove unsuccessful in a fractured rock environment. Contaminants can be transported great distances and at relatively high velocities along discrete channels in rock, so it is necessary to characterize both the rock matrix and properties of fractures that control or affect contaminant transport and remediation (NAS, 2020; ITRC, 2017).
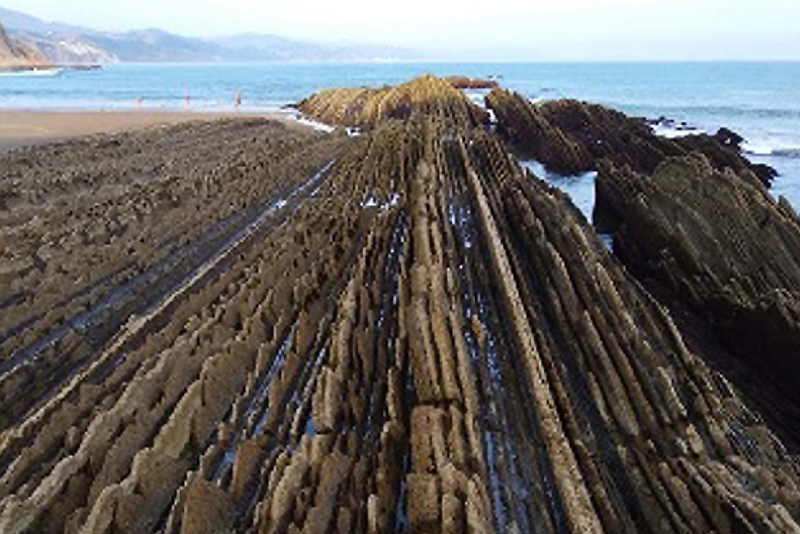
Outcrop of flysch (sequences of marl, shale, and sandstone) illustrating near-vertical bedding plan fractures in Zumaia, Spain. (Photo used with permission from B.G. Angel.
Rock formations can have both primary porosity (also called "matrix porosity") and secondary porosity. Primary porosity, the air-filled voids present when a rock forms (e.g., pore spaces between sand grains in a poorly cemented sandstone), is a function of the rock's texture. Secondary porosity develops after the rock has formed, either by fracturing (e.g., joints, faults, and bedding planes) or dissolution, such as the solution channels and cavities found in karst limestone. A poorly cemented sandstone can have a relatively high primary porosity that allows fluids to move through it. However, flow in fractured rock that has multiple fractures or fracture networks is affected by its secondary porosity which means fluid movement can be significant in rock formations with very low primary porosity, such as granite, if the rock has a network of interconnected fractures (ITRC, 2017).
Compared to unconsolidated deposits, significantly more uncertainty exists in fractured rock as to the direction and rate of contaminant migration, as well as the processes and factors that control chemical and microbial transformations (USGS, 2016). Depending upon the orientation and type of fractures, finding the contaminated zones and preferential pathways can be very challenging. Discrete fractures, both contaminated and uncontaminated, can be present in close proximity to each other resulting in potential risks of opening a conduit between fractures and fracture zones during borehole drilling.
The fate and transport of contaminants through fractured rock is a complicated process depending on the properties of the contaminants, the rock type, and the hydrology of the fractures. Mechanisms affecting fate and transport include (ITRC, 2017):
- Movement of contaminants through fractures
- Movement is controlled by four mechanisms: advection1, dispersion2, diffusion3, and capillary flow4.
- Matrix diffusion
- Dissolved contaminants can diffuse into a rock matrix with primary porosity and back out (back diffusion).
- Matrix diffusion can slow the rate of expansion of a contaminant plume5, but more importantly greatly increase the persistence of the plume even after sources have dissolved away or have been remediated. See the adjacent "Time Series Depiction of Cross Contamination and Rock-Matrix Diffusion in an Open Bedrock Borehole" animation for an example.
- Sorption and desorption
- Sorption of contaminants to rock surfaces or the rock matrix can slow down the migration of the plume and store contaminants for later release (desorption).
- Rocks with clay minerals and organic matter exhibit greater capacity to sorb contaminants.
- Natural attenuation through biotic and abiotic transformation
- Naturally occurring bacteria in groundwater can transform (e.g., reductive dechlorination of organic solvent) or a range of contaminants under the right conditions.
- Abiotic transformation includes chemical, oxidation, reduction, and electron transfer reactions.
- Volatilization
- Volatilization occurs in the unsaturated zone only.
- Contaminants volatilize into the unsaturated fractures or overburden soil.
When a non-aqueous phase liquid (NAPL) is introduced to a fractured rock environment, multiphase flow is particularly complicated as the dissolved-phase, NAPL-phase, and gas-phase flows can be different, even within the same fractured zone. In multiphase flow, density-based gravitational forces come into play along with capillary forces that act along the interfaces between the fluids (or gases) and the solid surfaces of the pores or fractures. The significance of these forces — gravity, viscosity, and capillary — vary with the pore size or fracture aperture, where capillarity dominates in smaller pores or fractures and gravity dominates in larger ones (Doe, 2010).
Despite the complexity of fractured rock flow, recent advances have improved characterization and remediation at fractured rock sites. This CLU-IN Fractured Rock focus area identifies resources that can help environmental practitioners with developing strategies for the characterization and remediation of fractured rock. For example, the 2017 guidance, Characterization and Remediation in Fractured Rock, by the Interstate Technology and Regulatory Council (ITRC) addresses "advances in skills, tools, and lessons-learned in understanding contaminant flow and transport in fractured rock environments." It covers geology, hydrogeology, the fate and transport of chemicals in a fractured rock environment, site characterization, remediation design, monitoring, and modeling in fractured rock environments (ITRC, 2017). Another resource is the 2020 Characterization, Modeling, Monitoring, and Remediation of Fractured Rock by the National Academy of Science (NAS, 2020) which examines "new developments, knowledge, and approaches to engineering at fractured rock sites" and builds upon the earlier 1996 National Research Council (NRC) report entitled Rock Fractures and Fluid Flow: Contemporary Understanding and Applications (NRC, 1996).
The Project Profiles page allows users to browse and search information (last updated 2010) on the nature and extent of the contamination at fractured bedrock sites and the actions taken to characterize and remediate the contamination. The focus area is periodically updated with new research papers and reports on demonstration and full-scale cleanups. If you know of a useful publicly available resource not currently listed, please suggest it for inclusion on the Suggest Resource page.
Bedrock Type
Knowledge of bedrock types and their typical properties is fundamental to understanding fractured rock aquifers. Bedrock types (igneous, sedimentary, and metamorphic) and the individual lithologies that occur within these groups directly influence primary porosity, secondary porosity, fracture characteristics (aperture, orientation, fabric, extent), and the physiography of an area or region and thus affect remedy selection (ITRC, 2017). The descriptions that follow summarize the primary and secondary porosity of various rock types. NAS (2005) designates fractured media as either a Type IV (Fractured Media with Low Matrix Porosity) or Type V (Fractured Media with High Matrix Porosity) setting; this designation is included in each description. For more information on bedrock types, see Appendix B of Characterization and Remediation of Fractured Rocks (ITRC, 2017).
Volcanic (Extrusive Igneous)
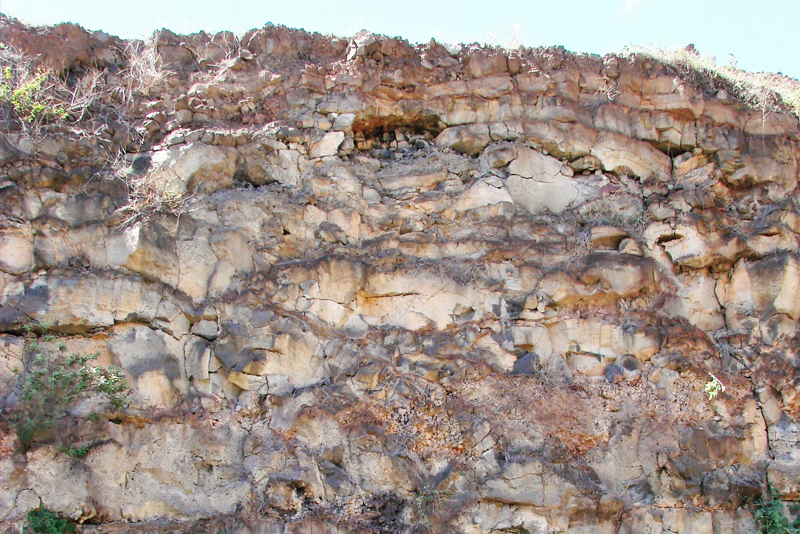
Pahoehoe flow lobe sequence belonging to the Koolau Basalt, visible at an old working face at a cement quarry in Hawaii. (Photo used with permission from Terry Tolan, INTERA.)
Unaltered pyroclastic6 deposits have porosity and permeability characteristics like those of poorly sorted sediments. If the ejected rock fragments were very hot as they settled, however, the pyroclastic material could become welded and almost impermeable. Silicic lavas, such as rhyolite or dacite, tend to be extruded as thick, dense flows and have low permeability except where they are fractured. Basaltic lavas tend to be fluid and can form extensive but thin flows that have a considerable amount of primary pore space (i.e., vesicles) at the tops and bottoms of the flows. Basalts are the most productive aquifers of all volcanic rock types (USGS, 1999). Extrusive rocks from lava or welded pyroclastic deposits would be classified as Type IV, in NAS's (2005) system. Contaminant transport in Type IV rock settings has only limited matrix diffusion effects, and secondary porosity caused by fracturing is the main transmissive feature (although fractures may or may not be connected). Extensively weathered crystalline rock, such as sometimes found at the top of bedrock, can exhibit more of a porous medium behavior.
Intrusive Igneous and Metamorphic
Spaces between the mineral crystals of intrusive igneous and metamorphic rocks are few, microscopically small, and generally unconnected. Consequently, the intergranular primary porosity of these crystalline rocks is so small as to be insignificant. Secondary fracture permeability in crystalline rocks is the result of the cooling of igneous rocks, deformation of igneous and metamorphic rocks, faulting, jointing, and weathering. Openings commonly are present along relict bedding planes, cleavage planes, foliation, and other zones of weakness in the rocks; these openings typically are heterogeneous in spacing, orientation, size, and degree of interconnection. Generally, openings in the rocks are most prevalent near land surface and decrease in number and size with depth (Olcott, 1995). The NAS (2005) describes intrusive igneous and metamorphic rocks as Type IV, which means contaminant transport has only limited matrix diffusion effects, and secondary porosity caused by fracturing is the main transmissive feature (although fractures may or may not be connected). Extensively weathered crystalline rock, such as sometimes found at the top of bedrock, can exhibit more of a porous medium behavior.
Sedimentary
Sandstone
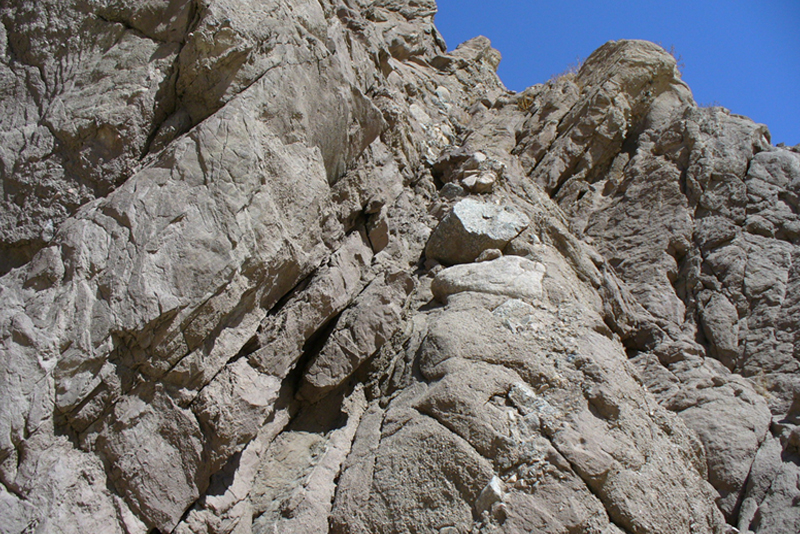
Fractured sandstone near the south end of the San Andreas Fault in California. (Photo used with permission from Ben Bentkowski, U.S. EPA Region 4.)
Sandstone aquifers are more widespread than other kinds of consolidated rocks. Although the porosity of well-sorted, unconsolidated sand may be as high as 50 percent, the porosity of most sandstones is considerably lower. During lithification, compaction by the weight of overlying material reduces the volume of pore space as the sand grains become more tightly packed, as well as the interconnection between pores (permeability). Cementation by calcite or silica between the sand grains further decreases porosity and permeability. Sandstones retain some primary porosity unless cementation has filled all the pores, but most of the porosity consists of secondary porosity openings such as joints, fractures, and bedding planes. Groundwater movement in sandstone aquifers primarily is along bedding planes, but the joints and fractures cut across bedding and provide avenues for the vertical movement of water between bedding planes (USGS, 1999). The NAS (2005) describes sandstone as Type V, which indicates contaminant transport is susceptible to matrix diffusion effects. A Type V setting includes systems where secondary porosity is the main transmissive feature and there is large void space in the matrix.
Shale, Claystone, Mudstone
These fine-grained rocks have low permeability, and fluid flow of any volume is through fractures, primarily along bedding planes. Their permeability is such, however, that dissolved contaminants may diffuse into the rock matrix, which can complicate groundwater cleanup efforts (USGS, 1999). The NAS (2005) describes Sandstone type hydrogeologic settings as a "Type V: Fractured media with High Matrix Porosity" where contaminant transport is susceptible to matrix diffusion effects. A Type V setting includes systems where secondary porosity is the main transmissive feature and where there is large void space in the matrix.
Limestone and Dolomite
The water-yielding properties of the carbonate rocks, limestone and dolomite, are highly variable. The primary porosity of the deposits can range from 1 to 40 percent (NAS, 2005) or more than 50 percent (USGS, 1999), depending on the degree of compaction, cementation, and dolomitization.
The principal post-depositional process that creates porosity in carbonate rocks is dissolution. Carbonate rocks are readily dissolved at depths shallower than about 300 feet below land surface where they crop out or are covered by a thin layer of material. Precipitation absorbs some carbon dioxide from the atmosphere and from organic matter in overlying soil, forming weak carbonic acid that dissolves the rocks. These small solution openings become larger, especially where a vigorous groundwater flow system moves the acidic water through the aquifer. Eventually, the openings join as networks of solution openings, some of which may be tens of feet in diameter and hundreds to thousands of feet in length. The end result of carbonate-rock dissolution is expressed at the land surface as karst terrane, characterized by caves, sinkholes, and other types of solution openings, and by few surface streams (USGS, 1999). Soluble rock alone is not sufficient to generate a karst terrane because these rock types often have low primary porosity that inhibits water infiltration and movement. For karst to develop, the introduction of groundwater through secondary porosity features, specifically fractures or bedding planes, is necessary (ITRC, 2017).
Where saturated, carbonate-rock aquifers with well-connected networks of solution openings yield large volumes of water to wells that penetrate the solution cavities, even though the undissolved rock between the large openings may be almost impermeable. Because water enters the carbonate-rock aquifers rapidly through large openings, any contaminants in the water can rapidly enter and spread through the aquifers (USGS, 1999).
Like other sedimentary rocks, carbonate rocks are classified as Type V in NAS's (2005) system. Karst settings differ, however, in that transmissive zones include solution openings that vary widely in aperture and have the potential to store and transport significant contaminant mass.
References:
U.S. Environmental Protection Agency (U.S. EPA), 2023. Time-Series Depiction of Cross Contamination and Rock-Matrix Diffusion in an Open Bedrock Borehole. Full Narrative Text.
Doe, T.W., Golder Associates Ltd. 2010. Fractured Bedrock Field Methods and Analytical Tools, Volume I: Main Report. Science Advisory Board for Contaminated Sites in British Columbia, University of Victoria, Victoria, BC, 99 pp, 2010.
Interstate Technology and Regulatory Council (ITRC), 2017. Characterization and Remediation of Fractured Rock. Web-based document FracRx-1.
National Academy of Science (NAS), 2020. Characterization, Modeling, Monitoring, and Remediation of Fractured Rock. National Academies Press, Washington, DC. ISBN: 978-0-309-37372-2, 176 pp, 2020.
National Academy of Science (NAS), 2005. Contaminants in the Subsurface: Source Zone Assessment and Remediation. National Academies Press, Washington, DC. ISBN: 978-0-309-09447-4, 370 pp, 2005.
National Research Council (NRC), 1996. Rock Fractures and Fluid Flow: Contemporary Understanding and Applications. National Academies Press, Washington, DC. ISBN-10: 0-309-10371-8, 568 pp, 1996.
Olcott, P., 1995. Ground Water Atlas of the United States: Connecticut, Maine, Massachusetts, New Hampshire, New York, Rhode Island, Vermont. U.S. Geological Survey, HA 730-M.
U.S. Geological Survey (USGS), 2016. Contamination in Fractured Rock Aquifers.
U.S. Geological Survey (USGS),1999. Ground Water Atlas of the United States: Introduction and National Summary Basaltic and Other Volcanic-Rock Aquifers, Carbonate-Rock Aquifers, Sandstone Aquifers.
Short, N.M., 2010. Remote Sensing Tutorial. Section 2: Concepts Underlying the Science of Geology in Remote Sensing Tutorial.
Resources
Fractures and Faults in Sandstone and Sandstone-Shale/Mudstone Sequences and Their Impact on Groundwater
The Groundwater Project, 2023
This book focuses on the properties most relevant to groundwater science such as permeability, porosity, as well as fracture length and frequency (density).
Introduction to Karst Aquifers
The Groundwater Project, 2022
The focus of this karst aquifer book is on water supply in formations composed of rocks that can dissolve (aquifers composed predominantly of carbonate and evaporite rocks).
Characterization and Remediation of Fractured Rock
Interstate Technology and Regulatory Council (ITRC), Web-based document FracRx-1, 2017
Contamination in Fractured Rock Aquifers
U.S. Geological Survey, 2016
USGS webpage lists research papers, fact sheets, and publications addressing contaminated fractured rock aquifers.
Characterization, Modeling, Monitoring, and Remediation of Fractured Rock
National Research Council. National Academies Press, Washington, DC. ISBN: 978-0-309-37372-2, 176 pp, 2020
Fractured Bedrock Field Methods and Analytical Tools, Volume I: Main Report
Doe, T.W., Golder Associates, Prepared for The Science Advisory Board for Contaminated Sites in British Columbia, 99 pp, 2010
Chlorinated Solvent Source and Plume Behavior in Fractured Sedimentary Rock from Field Studies
Parker, B.L.
NGWA/U.S. EPA Fractured Rock Conference: State of the Science and Measuring Success in Remediation, 24-26 September, Portland, Maine:150-168(2007)
The Effect of Matrix Diffusion on the Results of Large-Scale Tracer Experiments in a Fractured Dolomite (Abstract)
Novakowski, K.
NGWA/U.S. EPA Fractured Rock Conference: State of the Science and Measuring Success in Remediation, 24-26 September, Portland, Maine, 2007
Contaminants in the Subsurface: Source Zone Assessment and Remediation
National Academy of Science. National Academies Press, Washington, DC. ISBN: 978-0-309-09447-4, 370 pp, 2005
Matrix Diffusion-Derived Plume Attenuation in Fractured Bedrock (Abstract)
Lipson, D., B. Kueper, and M. Gefell.
Groundwater 43:30-39(2005)
Conceptual Models of Flow and Transport in the Fractured Vadose Zone
National Research Council. National Academies Press, Washington, DC. ISBN-10: 0-309-07302-8, 398 pp, 2001
Ground Water Atlas of the United States: Introduction and National Summary Basaltic and Other Volcanic-Rock Aquifers, Carbonate-Rock Aquifers, Sandstone Aquifers
U.S. Geological Survey,1999.
Rock Fractures and Fluid Flow: Contemporary Understanding and Applications
National Research Council. National Academies Press, Washington, DC. ISBN-10: 0-309-10371-8, 568 pp, 1996
Ground Water Atlas of the United States: Connecticut, Maine, Massachusetts, New Hampshire, New York, Rhode Island, Vermont
Olcott, P., U.S. Geological Survey, HA 730-M, 1995
Websites
USGS webpage that provides a general description of various aquifer types and where they are found in the United States.
Contamination in Fractured Rock Sites
USGS webpage that lists research, case studies, and publications by the USGS on characterizing and cleaning up fractured rock.
The website of DoD's SERDPESTCP environmental research program lists several documents that report the results of research on fractured rock characterization and remediation.
Helpful Definitions
Advection is the transfer of a contaminant by the flow of a fluid (either groundwater or the contaminant) due to bulk fluid motion under the influence of gravity or hydraulic pressure and gradients. Advection is the dominant transport mechanism in large-aperture fractures and is often the primary mode of contaminant transport under single-phase transport (dissolved phase) or multiphase transport (simultaneous water and NAPL, or water and gas migration). ↩
Advection is the transfer of a contaminant by the flow of a fluid (either groundwater or the contaminant) due to bulk fluid motion under the influence of gravity or hydraulic pressure and gradients. Advection is the dominant transport mechanism in large-aperture fractures and is often the primary mode of contaminant transport under single-phase transport (dissolved phase) or multiphase transport (simultaneous water and NAPL, or water and gas migration). ↩
Dispersion is the spreading of dissolved chemicals due to the combined effects of mechanical mixing and diffusion (ITRC, 2017). The transport rate and flow direction of the contaminants is not necessarily the same as the groundwater. The contaminant spreads laterally and vertically depending on rock fracture and matrix geometries as well as local variations in flow velocity (NRC, 2020), so mixing occurs along the flow path. ↩
Dispersion is the spreading of dissolved chemicals due to the combined effects of mechanical mixing and diffusion (ITRC, 2017). The transport rate and flow direction of the contaminants is not necessarily the same as the groundwater. The contaminant spreads laterally and vertically depending on rock fracture and matrix geometries as well as local variations in flow velocity (NRC, 2020), so mixing occurs along the flow path. ↩
Diffusion is the movement of dissolved contaminants from areas of higher chemical concentration to areas of lower chemical concentration. Diffusion within fractures is more significant over shorter distances (such as centimeters to meters). Contaminant diffusion can play a major role in contaminant migration in porous media with low hydraulic conductivity. For example, shale is moderately impermeable to water flow through the matrix, but still can have sufficient porosity to allow for the diffusion of contaminants into the matrix (Vitolins et al. 2004). ↩
Diffusion is the movement of dissolved contaminants from areas of higher chemical concentration to areas of lower chemical concentration. Diffusion within fractures is more significant over shorter distances (such as centimeters to meters). Contaminant diffusion can play a major role in contaminant migration in porous media with low hydraulic conductivity. For example, shale is moderately impermeable to water flow through the matrix, but still can have sufficient porosity to allow for the diffusion of contaminants into the matrix (Vitolins et al. 2004). ↩
Capillary flow is the flow of a contaminant into narrow fractures without the assistance of, and often in opposition to, gravity. This type of fracture flow can be important in the movement of NAPL and an important consideration in remediation. ↩
Capillary flow is the flow of a contaminant into narrow fractures without the assistance of, and often in opposition to, gravity. This type of fracture flow can be important in the movement of NAPL and an important consideration in remediation. ↩
"Plume" does not precisely apply to a zone of contaminated groundwater in bedrock. For example, a plume may be a narrow area of contamination moving within a transmissive fracture (ITRC, 2017). ↩
"Plume" does not precisely apply to a zone of contaminated groundwater in bedrock. For example, a plume may be a narrow area of contamination moving within a transmissive fracture (ITRC, 2017). ↩
Pyroclastic Refers to materials (from ash to large fragments of rock) ejected from a volcano during an eruption. ↩
Pyroclastic Refers to materials (from ash to large fragments of rock) ejected from a volcano during an eruption. ↩