- Featured Articles
- Emerging Technologies: 1,4-Dioxane Occurrences and Treatment Options in Private Wells
- Treatability Studies: Using Ferns for Arsenic Bioaccumulation at Georgia Superfund Site
- Resources
Download This Issue in Adobe PDF® Format
(600KB/8pp/PDF)
This issue of Technology News & Trends highlights technologies for characterizing and remediating sites with persistent organic pollutants (POPs) such as polychlorinated biphenyls (PCBs), dioxin/furans, and certain pesticides. A total of 22 POPs are now recognized by the Stockholm Convention as having the properties of:
- Remaining intact for exceptionally long periods of time
- Becoming widely distributed throughout the environment
- Accumulating in the fatty tissue of living organisms, and
- Posing toxicity threats to humans and wildlife.
Historically, cleanup of soil, sediment, and groundwater contaminated with POPs commonly involved excavation, dredging, or ex situ thermal processes followed by offsite disposal. Use of these technologies can result in significant project costs, landfill burdens, potential dispersion of contaminants, and/or combustion-related creation of carcinogens. New alternatives undergoing tests in laboratory or field settings often involve bioremediation, plant-based, or oxygenation technologies.
FEATURED ARTICLES
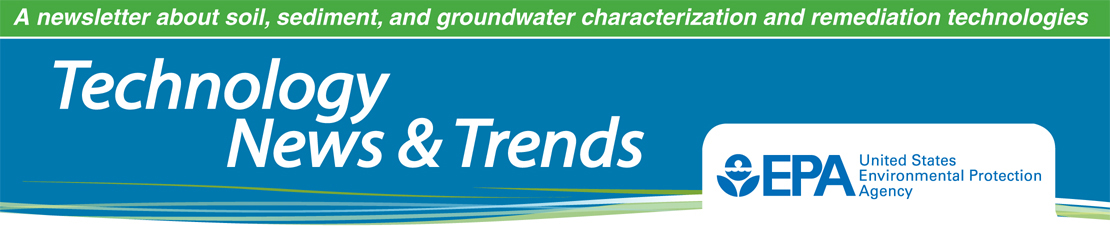
Emerging Technologies: 1,4-Dioxane Occurrences and Treatment Options in Private Wells
Contributed by Thomas K.G. Mohr*
1,4-Dioxane is a persistent organic pollutant that is highly mobile and tends to form long plumes significantly advanced from the leading edge of a chlorinated solvent plume, and its detection is often missed during site investigation and plume delineation. Consequently, many monitoring well networks, capture zones, and treatment trains are designed to characterize, recover, and remove chlorinated solvents but not associated 1,4-dioxane contamination. Certain volatile organic compounds (VOCs) such as trichloroethane (TCA) are often co-contaminants of 1,4-dioxane and may be used to trigger consideration of 1,4-dioxane's extreme mobility and whether testing of private wells far beyond the extent of the VOC plume is warranted. Results of a 2006 U.S. Geological Survey study of 1,208 domestic wells, as summarized in Volatile Organic Compounds in the Nation's Ground Water and Drinking-Water Supply Wells, detected the presence of 1,1,1-TCA, 1,1-dichloroethane, and 1,1-dichloroethene (as potential 1,4-dioxane co-contaminants) in as many as 102, 26, and 19 wells, respectively.
1,4-Dioxane in groundwater is resistant to natural biodegradation or treatment technologies such as air stripping, carbon adsorption, reverse osmosis, and ion exchange. Advanced oxidation technologies employed ex situ (such as ozone peroxidation, ultraviolet-oxidation, and catalyzed photo-oxidation) often are used for this purpose but carry a high capital and operating cost. For owners of private wells affected by contaminated sites, these costs are prohibitive. Alternatives such as connecting to a municipal supply or exclusively using bottled water also may be costly or infeasible.
Granular activated carbon (GAC) filters typically have been used to treat chlorinated solvents in domestic supply wells and are now being used in an attempt to treat 1,4-dioxane simultaneously. Although GAC is not expected to perform well for 1,4-dioxane removal due to its low organic carbon partitioning coefficient (KOC), in some cases the retention rates in GAC have exceeded the calculated sorption rates, suggesting that matrix diffusion by 1,4-dioxane into the carbon structure is a potential removal mechanism. Due to its anomalously high dipole moment in aqueous solutions, 1,4-dioxane is an efficient water-structure breaker capable of penetrating the tightly held monolayer of water on clay surfaces, and may similarly penetrate activated carbon's porosity in a way that more hydrophobic non-polar contaminants cannot.
Adaptations for deploying GAC in 1,4-dioxane removal have proven more effective than expected at several sites where small privately-owned wells are used for residential or industrial purposes. Successful methods for employing GAC technology have included installing conventional well carbon cartridges in series with frequent midpoint sampling and frequent lead vessel change-out; operating at flow rates substantially lower than the carbon vessel flow capacity; and using substantially over-sized carbon vessels operating in series.
For example, in the Tylerville section of Haddam, Connecticut, two 3-cubic-foot carbon vessels were installed in series on a well with 10-20 micrograms per liter (µg/L) 1,4-dioxane as part of remedial investigations under the Connecticut Department of Energy and Environmental Protection (CTDEEP) Superfund Program. Breakthrough of 1,4-dioxane was experienced after four and nine months, prompting frequent replacement of the carbon vessels despite their continued removal of targeted TCE and other chlorinated solvents. Purchase and installation costs for a typical GAC filter system in this type of setting average approximately $2,500, with single filter replacements costing about $700.
CTDEEP field experience at Tylerville and other sites found that 1,4-dioxane concentrations in mid-point and end-point samples of water lines are occasionally higher than in influent samples, suggesting buildup and subsequent release of 1,4-dioxane (sloughing). This finding is consistent with previous research by others, which suggests that the observed breakthroughs at the Tylerville well were due to the carbon absorption and adsorption reaching their capacity for retaining 1,4-dioxane, or to a "chromatographic effect." i.e., initial adsorption followed by displacement by compounds with stronger affinity to adsorb to carbon.
Absorption of 1,4-dioxane into GAC pores, as opposed to adsorption onto GAC surfaces, is among the possible mechanisms for complete removal of 1,4-dioxane in groundwater at the U.S. Department of Energy's Stanford Linear Accelerator Complex (SLAC) in California. The treatment process's very slow flow rate (0.2 gallons per minute (gpm)) coupled with a 106-cubic-foot capacity in each GAC vessel (634 gallons in both vessels, accounting for a 40% void volume) leads to a slow bed-volume exchange (0.45 bed volumes per day) and a long contact duration (53 hours of transit time through the two GAC vessels). The grade of carbon selected for VOC removal is a coconut shell-based GAC (AquaCarb® 1230C) designed to remove poorly adsorbable organics from water such as MTBE and other trace-level organics. This product is an acid-washed pH-neutral GAC with a low ash content designed for use in potable water systems and in high-purity water systems for the microelectronics and other industries. It also passes the ANSI/NSF Standard 61 for use in potable water applications.
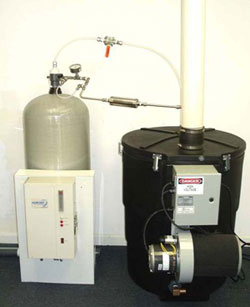
Figure 1. Single in-line water treatment system involving both ozone/perozone injection and air stripping technologies.
To remove 1,4-dioxane in smaller private wells, one option is an advanced oxidation system known as ClearDioxane™. The system uses a micro-bubbler and ozone generator to inject ozone and perozone (peroxide-coated micro- to nano-bubble ozone) into a pressurized hydrodynamic tank to achieve mixing and optimal residence time (Figure 1). The tank discharges to a modified shallow-tray air stripper (the Clearadon™ stripper) for ozone and VOC removal, and the treated water is then repressurized for delivery within the water line.
Preliminary results from bench-top testing of the oxidation system show a significant reduction in 1,4-dioxane concentration, from 100 µg/L 1,4-dioxane to less than 3 µg/L. Follow-up field trials are underway in New Jersey and Florida. The cost to equip a 10-gpm well with this system is estimated at $18,000-$20,000. Its operating cost is strongly driven by electricity consumption. Application for a 6- to 10-gpm well, for example, may involve an energy demand of 20 kilowatts/hour (kWh). If deployed at a site where electricity costs $0.12/kWh, the cost to operate a system of this size is estimated at $2.40 for each 1,000 gallons of water treated. While prohibitive in many cases, these costs may be necessary in rural areas where hookup to a municipal water supply is infeasible.
Using GAC or the advanced oxidation system as a point-of-entry treatment (POET) system for private wells is preferred over a point-of-use treatment (POUT) system installed on individual faucets, since a POET system is installed on the primary water intake valve that routes treated water to all connected faucets. Most POET systems, however, are designed to treat only specific contaminants rather than all contaminants or potentially harmful bacteria and consequently cannot be used for all-purpose filtration.
Most state health agencies do not issue operating permits for small private well systems, and securing an independent certification of a well-water treatment system can involve a lengthy process. Additional case studies and information exchange are needed to help cleanup decision-makers better understand the fate and transport of 1,4-dioxane and implement reliable treatment technology for affected private wells. More information on 1,4-dioxane can be obtained from the U.S. Environmental Agency's Treatment Technologies for 1,4-Dioxane: Fundamentals And Field Applications report and the 1,4-Dioxane and Other Solvent Stabilizers White Paper available from the Santa Clara Valley Water District.
* Thomas K.G. Mohr is a past President of the Groundwater Resources Association of California and author of "Environmental Remediation and Investigation: 1,4-Dioxane and Other Solvent Stabilizers" (CRC Press, 2010). Mr. Mohr is currently employed by the Santa Clara Valley Water District and independently pursues the 1,4-dioxane challenge.
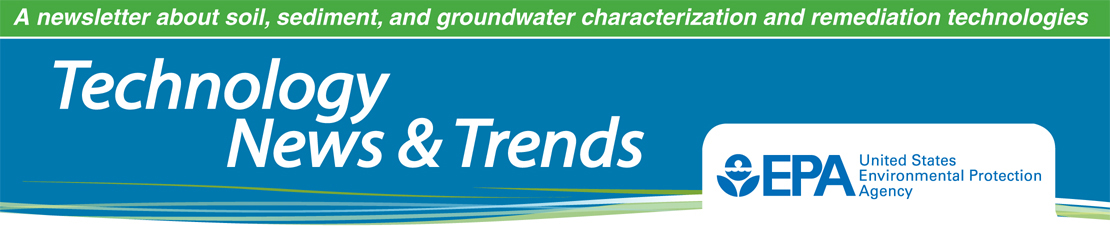
Treatability Studies: Using Ferns for Arsenic Bioaccumulation at Georgia Superfund Site
Contributed by Charles King and Linda George, U.S. EPA Region 4; and Joe Owusu-Yaw, Ph.D., ILS
The U.S. EPA Region 4 office recently completed a treatability study on the use of ferns to remediate arsenic contamination at the 31-acre Woolfolk Chemical Works, Inc. Superfund site in Fort Valley, Georgia. At various times from the 1910s to the early 1980s, approximately 18 acres of this site were used to manufacture arsenic trichloride as well as pesticides, insecticides, and herbicides such as DDT, lindane, and toxaphene. Removal and remediation actions initiated in 1984 have involved soil excavation and offsite disposal, groundwater extraction and treatment, and/or installation of lined containment cells across four operable units.
The recent treatability study supports remedy selection for a fifth operable unit (OU5) associated with offsite drainage potentially affecting adjacent commercial or residential neighborhoods and approximately three miles of watershed downstream. The study involved bench-scale tests using native ferns and non-native ferns that are known to hyperaccumulate arsenic in downstream wetlands and riparian buffers. Selected fern species also were tested under greenhouse conditions.
Initial activities involved collecting surface soil or sediment samples from five stations at OU5 in 2009. To confirm that the collected samples provided a range of arsenic concentrations suitable for treatability testing, x-ray fluorescence was used to screen dried samples prior to analysis at EPA Region 4's Science and Ecosystem Support Division (SESD) laboratory. Analytical results showed arsenic concentrations in soil ranging from 22 mg/kg to 180 milligrams per kilogram (mg/kg) dry weight (dw) with a pH of 3.52 to 5.07, below the optimum pH 6-7 needed for arsenic extraction.
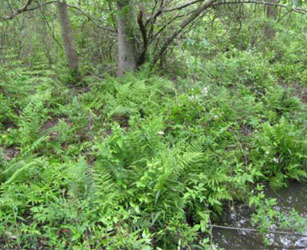
Figure 1. Diverse fern community at a reference station near the Woolfolk Chemical Works, Inc. site.
Several species of native ferns existing along a downgradient creek and in adjoining floodplains also were collected for analysis. Observed species included ebony spleenwort (Asplenium platyneuron), wood ferns (Dryopteris sp.), netted chain fern (Woodwardia areolata), bracken fern (Pteridium aquilinum), cinnamon fern (Osmunda cinnamomea), royal fern (Osmunda regalis), and sensitive fern (Onoclea sensibilis) (Figure 1). The fern samples were analyzed at an offsite Contract Laboratory Program (CLP) facility using inductively coupled plasma (ICP) atomic emission spectroscopy. Results indicated arsenic concentrations were highest in ebony spleenwort (30 mg/kg dw with a co-located soil concentration of 180 mg/kg dw).
Two bench-scale studies were conducted at the SESD laboratory. The studies focused on three fern species expected to have the greatest accumulation potential for this site: Cretan brake (Pteris cretica 'Mayi') and ladder brake (Pteris vittata) available from a bioremediation specialty firm, and native ebony spleenwort obtained from a local supplier. Using soil/sediment collected from the five sampling stations, the ferns were grown under ambient laboratory illumination of 1,000-1,500 lux at a temperature of 21�2°C for up to seven weeks with a photoperiod of 16 hours of light and 8 hours of darkness. Following growth under various experimental conditions, tissues of fern replicates for each soil/sediment station were submitted to a CLP laboratory for analysis of arsenic content (uptake).
The first bench-scale study focused on arsenic uptake of the cretan brake fern. The uptake was relatively negligible over seven weeks, except in ferns growing in soil collected from the station with the highest arsenic concentration (180 mg/kg dw); ferns from this single station had accumulated 600 mg/kg dw of arsenic (Figure 2). The maximum bioaccumulation factor was estimated at 3.33. This relatively high arsenic uptake in the fern was attributed to the soil's higher arsenic concentrations, lower moisture content, and sandier texture (which can allow more aeration). Previous studies by others suggest that these conditions would better promote tissue uptake of arsenic in the form of arsenate (arsenic V) rather than arsenite (arsenic III), which can be reduced to arsenite and subsequently sequestered in the fronds.
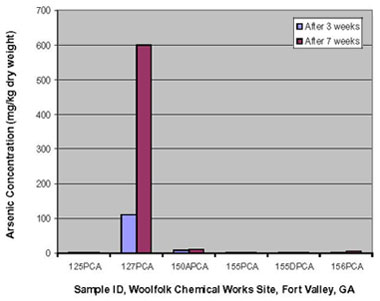
Figure 2. Arsenic accumulation in the fern Pteris cretica 'Mayi' during bench-scale studies.
Similar conditions were used in the second bench-scale study, which was performed on ladder brake fern and ebony spleenwort. In addition, lime or fertilizer was added to some samples to determine if such amendments would enhance arsenic uptake. The ladder brake ferns did not grow as expected, possibly due to transplanting shock, and achieved little to no arsenic uptake; the maximum concentration uptake for arsenic was 26 mg/kg dw. Growth of the three-year-old ebony spleenwort was more robust, resulting in a maximum arsenic uptake of 39 mg/kg dw (Figure 3), which was slightly higher than the 30 mg/kg dw concentration earlier found in ebony spleenwort growing naturally at the same field station. Adjusting the soil pH to neutral or fertilizing the plants had little or no effect on arsenic uptake in this study.
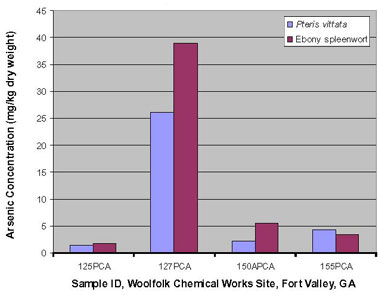
Figure 3. Arsenic accumulation in the ferns Pteris vittata and ebony spleenwort during bench-scale studies.
Researchers at the nearby Fort Valley State University (FVSU) conducted the companion greenhouse tests in 2011, using Woolfolk soil (or sediment) associated with differing onsite drainage conditions and arsenic concentrations ranging up to 150 mg/kg. Testing focused on the ladder brake fern as well as silver ribbon fern (Pteris cretica 'Albo-lineata'), which are both hardy perennials that can be harvested up to three times in a six-month period. Based on the results of routine soil analysis performed by the University of Georgia's Agricultural and Environmental Services Laboratories, lime was added to the experimental pots to raise soil pH to levels needed for optimal fern growth. Soil treatments involved individual six-inch pots containing either ladder brake fern, silver ribbon fern, ladder brake fern with triple superphosphate, silver ribbon fern with triple superphosphate, or soil with triple superphosphate but no fern.
Both species of fern were observed to flourish in the Woolfolk soil under greenhouse conditions, regardless of the soil moisture or arsenic content. Four of the eight treatment pots and two of the four control pots were sacrificed after six weeks of plant growth, and the remaining were sacrificed after 13 weeks. Samples of the fern fronds, roots, and soil were separately composited and submitted to the EPA Region 4 SESD laboratory for analysis. Analytical results indicated arsenic reductions in soil reached 26.8%, although higher removal rates are expected in more mature plants during a normal growing season. The ladder brake fern and silver ribbon fern accumulated arsenic at similar rates with no apparent influence from phosphorus amendment. In addition to supporting remedy selection for OU5, results of the greenhouse tests are being integrated into broader phytoremediation studies led by Cornell University and the University of Maryland.
Based on final results of the SESD treatability study and FVSU greenhouse tests, EPA Region 4 will conduct an onsite pilot-scale field project if funding is available. The project would rely on ladder brake, cretan brake, or silver ribbon ferns as the arsenic hyperaccumulators and may involve plant fertilization, irrigation, soil enhancements such as aeration and pH adjustment, and/or soil amendments.
RESOURCES
CLU-IN Website
- POPs: treatment technology reports
- PCBs: an overview of aspects such as toxicology, detection and site characterization, and treatment technologies
- 1,4-Dioxane: an overview
State Initiatives: California Biomonitoring Program
Under this new program, the State of California will measure the toxic chemicals accumulating in the bodies of Californians. The measurements will provide a snapshot of which chemicals in Californians are rising, which are falling, and which are emerging chemicals of concern (including POPs). The California Department of Toxic Substances Control will use information on the levels of chemicals in humans and wildlife to identify problem chemicals, understand where to focus pollution prevention efforts, prioritize chemical cradle-to-cradle efforts, and use these chemicals as indicators to measure success of state interventions.
EPA developed this user guide to provide a consistent approach for using incremental and compositing techniques in assessment of dioxin in shallow soil. A companion template is available to use as a platform for compiling site-specific and technical background information.
Contact Us:
Suggestions for articles in upcoming issues of Technology News and Trends may be submitted to
Linda Fiedler via email at fiedler.linda@epa.gov.
Past Issues:
Past issues of the newsletter are available at http://www.clu-in.org/products/newsltrs/tnandt/.
Archives | Subscribe | Change Your Address | Unsubscribe